Written by: Jenna Kerry, MSc
Published: February 2, 2024
Introduction
Once antibody discovery, lead selection, and characterization is complete, researchers have a range of expression systems to choose from to produce the antibody of interest. When choosing an expression system, researchers have to consider many factors such as protein complexity, post-translational modifications (PTMs), and downstream applications. This blog will delve into the most commonly used recombinant expression systems, exploring their nuances, benefits, and drawbacks.
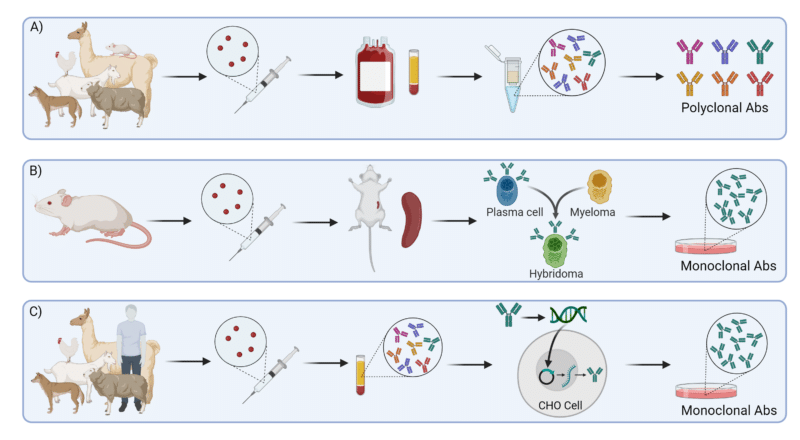
Figure 1: Overview of Antibody Production Methods A) Animal immunization, B) Hybridoma Technology, and C) Recombinant Expression System.
Recombinant Expression Systems
Mammalian Expression System
Widely employed in the pharmaceutical industry for the production of recombinant monoclonal antibodies (mAbs) utilized in therapies, diagnostics, and biomedical research, mammalian expression systems showcase distinctive advantages (Table 1). Mammalian cells serve as the preferred hosts for production of mammalian antibodies because they are more likely to produce desirable post-translational modifications (PTM). These modifications ensure correctly folded and glycosylated proteins, thereby influencing antibody binding affinity, stability, and effector functions.
Cell lines derived from various species, such as humans (HEK293), hamsters (CHO), and mice (NS0), can be utilized to establish mammalian expression systems. However, over 70% of currently approved biotherapeutic proteins are produced in Chinese hamster ovary (CHO) cells. This preference is attributed to several factors. Importantly, CHO cells efficiently grow in cell suspension, displaying high productivity and adaptability, facilitating large-scale industrial culturing. Additionally, CHO cells can thrive in serum-free chemically defined media, contributing to enhanced batch-to-batch reproducibility. CHO cells also demonstrate low susceptibility to human viruses, ensuring their safety for use in human therapeutics. Lastly, CHO cells exhibit a remarkable capacity for genetic manipulation, enabling the expression of desired traits.
While using CHO cell lines for recombinant antibody production offers numerous advantages, it has drawbacks. One notable challenge is that CHO cells have an inherently plastic genome, leading to unforeseen genomic rearrangements that may pose challenges for early production. Furthermore, CHO cells cannot produce all human glycosylation patterns, as they lack specific sialyltransferases and fucosyltransferases. They also produce glycans not expressed in humans, such as α-gal and NGNA, which could induce immunogenicity or impact effector function. For these reasons, there have been efforts to engineer CHO cell lines to produce more favorable glycoforms
Alternatively, human cell lines can be used which exclusively produce proper human PTMs, reducing the cost of additional processing and, importantly, eliminating the immunogenicity of nonhuman glycans. For example, human embryonic kidney 293 cells (HEK293) produce proper human PTMs while also being easily scalable to large scale production and adaptable to growth in suspension with serum-free chemically defined media. However, HEK293 cells are susceptible to infection with human viruses, necessitating careful consideration of safety and efficacy for use in human therapeutics.
Bacterial Expression Systems
Bacteria offer several advantages for protein expression, including straightforward culture techniques, simple media requirements, and high protein production capacity (Table 1). Moreover, protein expression in bacteria can be regulated by lactose through the use of a promoter. However, their simplicity poses challenges for complex PTMs, potentially impacting antibody function. As a result, bacterial expression systems are typically not used to express full mAbs, however, they can be used to produce antibody subunits that do not need to be glycosylated.
Various bacteria can be employed for recombinant protein production, with E. coli and B. subtilis being popular choices. E. coli is favored for its ease, speed, and cost-effectiveness, with a growth rate as short as 20 minutes and continuous fermentation capabilities. Moreover, E. coli has many commercially available expression vectors making them simple and versatile.
Despite the advantages of E. coli, it has notable drawbacks. E. coli lacks the cellular machinery for glycosylation, phosphorylation, sulfitation, and disulfide formation which are crucial for proper protein function, folding, and stability. Incorrect protein folding can lead to the formation of inclusion bodies within bacteria due to the absence of a protein chaperone system. In such cases, proteins must be extracted from the inclusion bodies and refolded. Moreover, E. coli may accumulate endotoxins, posing challenges for applications in human therapeutics. As an alternative, B. subtilis is sometimes chosen for its lack of harmful endotoxins. However, B. subtilis has its drawback – the production of an extracellular protease that can degrade the protein of interest.
Yeast Expression Systems
Yeast expression systems strike a balance between mammalian and bacterial systems, capable of performing some PTMs while being more cost-effective than mammalian cells (Table 1). This well-characterized and biosafety-compliant platform has been extensively utilized to express various proteins for vaccines, pharmaceutical products, and diagnostics. Notably, yeast has successfully contributed to the development of vaccines, including those targeting the hepatitis B virus and human papillomavirus. An advantageous feature of yeast is its endomembrane system, facilitating the secretion of expressed proteins into the extracellular environment. Moreover, yeast produces properly folded soluble recombinant proteins, incorporating functional PTMs. The ease of manipulating the yeast genome further enhances its production capabilities compared to mammalian counterparts.
However, much like the other expression systems, yeast expression systems have significant drawbacks. While capable of performing some complex glycosylation, they exhibit slight differences in the formation of N and O-linked glycosylation compared to mammalian cells. Particularly, S. cerevisiae cannot precisely mimic mammalian glycosylation and tends to hyper-glycosylate N and O-linked glycan structures within proteins. For achieving proper glycosylation patterns, the preference leans towards P. pastoris, as its glycosylation more closely resembles mammalian counterparts. Ongoing efforts by researchers aim to develop glycoengineered yeast with fully humanized sialylated glycoproteins, addressing concerns related to hyper-glycosylation. Despite these limitations, yeast remains suitable for producing antibodies with moderate glycosylation requirements, commonly adopted in research-scale or diagnostic antibody production.
Plant Expression Systems
Plant expression systems have recently gained attention over the other conventional systems listed above. With the recent advancements, plants have gained the ability to produce potent neutralizing antibodies at a very high yield that are proven to have therapeutic effects. On top of being incredibly cost-efficient, simple, and scalable, plant expression systems are also capable of producing antibodies with eukaryotic PTMs and folding (Table 1).
Plants have already been used to produce several mAb to treat diseases such as HIV, West Nile virus, and rabies that are on the way to commercialization. Additionally, plants can be grown in a field making production very inexpensive, and scale-up is much easier than other expression systems. However, since they are grown in a field, plant expression systems are subjected to variations in product yield, contamination, and susceptibility to pests and diseases. Moreover, produced recombinant proteins can be stored in the leaves and seeds leading to difficult downstream protein isolation.
Insect Expression Systems
Insect expression systems have been used since the mid-1980s with the most popular being the baculovirus expression vector system (BEVS). Baculovirus can insert the protein of interest into insect cells without being hazardous for humans. Insect cells can generate mammalian PTMs, but have inconsistencies in glycosylation patterns leading to changes in biological function. Insect expression systems are advantageous due to the ease of culture conditions and the ability for production of a broad range of proteins simultaneously. However, since the baculovirus infection ends with cell death, the recombinant proteins have to be produced in batches leading to inconsistencies (Table 1).
Table 1: Summary of the advantages and drawbacks of the recombinant expression systems.
Recombinant Expression of Polyclonal Antibodies
In contrast to monoclonal recombinant expression systems, the native immune response uses complex polyclonal antibodies (pAbs) in order to effectively fight disease. Animal immunization is widely used to produce pAbs that have naturally undergone affinity maturation. Evidence has accumulated that suggests a hybrid approach between animal immunization and recombinant expression systems is the best option to capture the benefits of each method. This hybrid approach, namely polyclonal recombinant expression, combines the broad-spectrum coverage of pAbs with the consistency and safety of recombinant mAbs. In fact, a recent case study found that the polyclonal recombinant antibodies (rAbs) performed just as well or better than the original pAbs. The process to generate polyclonal rAbs is as follows (Figure 2):
Figure 2: Overview of Recombinant Polyclonal Antibody Generation using the REpAb De Novo Sequencing Platform.
- Animal Immunization: An animal is immunized with the antigen of interest which triggers an immune response that generates pAbs. The blood from the immunized animal is extracted, and the serum is purified using antigen purification to isolate the pAbs of interest.
- Sequence pAbs via REpAb: The isolated pAbs are digested into peptides using a multi-enzyme digest. These peptides are then sequenced using liquid chromatography with tandem mass spectrometry (LC-MS/MS) and full antibody sequences are generated de novo using machine-learning (ML)-based bioinformatics.
- mAb Cocktail Recombinant Production: The pAb antibody sequences are each inserted into their own expression vector to generate a panel of mAb expression vectors. This is followed by pilot-scale transient expression in the cell line of choice (see above) for each mAb.
- pAb Characterization: The produced mAbs undergo different purification steps, such as affinity chromatography, to remove impurities and isolate the mAbs of interest. After purification, the antibody or nanobody can be put through different quality control measures, such as SDS-PAGE, enzyme-linked immunosorbent assay (ELISA), and sequence confirmation by mass spectrometry.
Antibody Sequencing and Antibody Characterization Services
Regardless of which recombinant expression cell line you choose, you must ensure the production of accurate, reliable, high-quality antibodies that are specific and perform the intended function.
- Our full suite of antibody characterization services can identify post-translational modifications (PTMS), sequence variants, and disulfide linkages for early production pipelines.
- Our glycan analysis service via LC-MS can help you explore the differences between wild type, recombinantly expressed and engineered mAbs, to more fully understand binding and performance
- MATCHmAb peptide mapping service provides a fast and economical way of checking that your recombinant/engineered mAb was produced correctly
- RapidSPR uses surface plasmon resonance to help you screen, select and profile your mAbs and explore the differences in performance caused by sequence and glycan modifications
- Antibody sequencing service or antibody discovery services to de novo antibody sequencing are an ideal starting point for those wishing to derive the sequences of monoclonal or polyclonal antibodies respectively.
To explore how Rapid Novor can enhance your engineering, production and quality control processes, contact our scientists today!
Talk to Our Scientists.
We Have Sequenced 10,000+ Antibodies and We Are Eager to Help You.
Through next generation protein sequencing, Rapid Novor enables reliable discovery and development of novel reagents, diagnostics, and therapeutics. Thanks to our Next Generation Protein Sequencing and antibody discovery services, researchers have furthered thousands of projects, patented antibody therapeutics, and developed the first recombinant polyclonal antibody diagnostics.
Talk to Our Scientists.
We Have Sequenced 9000+ Antibodies and We Are Eager to Help You.
Through next generation protein sequencing, Rapid Novor enables timely and reliable discovery and development of novel reagents, diagnostics, and therapeutics. Thanks to our Next Generation Protein Sequencing and antibody discovery services, researchers have furthered thousands of projects, patented antibody therapeutics, and ran the first recombinant polyclonal antibody diagnostics